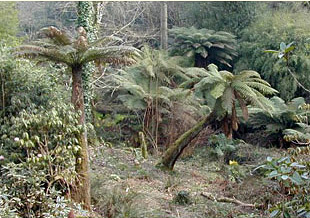
By the ploughshare, willingly gave everything.
In Genesis the earth needs to be cultivated from the start. It differed only in its physical structure. Though the writer had no scientific knowledge to guide him and it might be argued that he made the account up, the differences are consistent with his affirmation that the primeval landmass was destroyed, and that the landmass that took its place was fundamentally new.
Rain originally was not part of the natural order (Gen 2:5). The world was watered by moisture that oozed through the land mass from a reservoir beneath (2:6), called the ‘deep’ (7:11, 8:2) (Tsumura 1989). If oceans had surrounded the land, evaporation would have generated clouds (as the Hebrews knew – Job 36:27f), and the clouds would have produced rain, but Genesis does not say there were great surface oceans. It says that the waters were gathered ‘into one place’, as a result of which dry land emerged. The gathered waters were called (by the one who brought them into being) ‘seas’ and occupied one location because they were all beneath the land. Psalm 24 says explicitly that the land was founded upon seas and rivers. ‘Founded,’ yasadah, is a building term. The land was pictured as resting on pillars sunk into a single, subterranean ocean which was thereby partitioned into ‘deeps’, plural. Such seas or great lakes as existed on the surface would have got their waters from the deep. Rivers likewise. Rainbows and clouds were new phenomena in the post-Flood world (9:13f) because rain was a new phenomenon. The ocean from which moisture could evaporate was now at the surface. A different water cycle had come into operation.
Here, then, is evidence that the details of Genesis 1–6 were not the product of speculation, based on what Israel inferred about the present world. In ancient Palestine, as in any agricultural society, rain was of supreme importance, since it was the source of the water needed for growing crops and sustaining the pastures that fed their animals. Egypt had the Nile, Mesopotamia the Tigris and Euphrates. Canaan had no major rivers other than the Jordan along the eastern edge of the country. It was a land of wadis and underground springs (Deut 8:7), drinking irregularly from the rain of heaven (Deut 11:11). If the rains did not come, the result was drought and famine. It is significant therefore that the only mention of rain falling in Genesis’s description of the first world is when that world comes to an end (7:4).
Far from the Israelites basing their picture of the antediluvian world on their perceptions of the present one, the reverse seems to have been true. They described the present world as if it was largely unchanged since antediluvian times. Jacob promised his son Joseph that he would receive blessings of heaven above and ‘blessings of the deep that couches beneath’. A psalm reminds the Israelites that the rocks which God cleft in the wilderness gave them drink ‘as from the great deep’, and Ezekiel says of a Lebanese cedar that ‘the deep made it grow tall, making its rivers flow round the place where it was planted” (31:4). Fish lived ‘in the water under the land’ rather than simply ‘in the sea’.
and all their host by the breath of his mouth.
He gathered the waters of the sea as a heap;
he put the deeps in storehouses.
‘As a heap’ is the equivalent of Genesis’s ‘in one place’, and his putting the sea ‘in storehouses’ a metaphorical way of saying that he went on to divide its mass into separate bodies or pockets of water (we too commandeer metaphors – ‘bodies’, ‘pockets’ – to make language serve). The psalmist was alluding to a tradition which was part of the nation’s heritage, which psalmists themselves helped to maintain (Job 36:24). Their works were poetic inasmuch as their language was elevated, as befitted prayers, laments, declarations of praise, meditations, but the things they spoke about were factual. When Solomon elaborately retold the acts of creation in the midst of his proverbs, he too may have been drawing on tradition.
The Hebrew word for the ‘deep’ was tehom. The city-state of Ugarit, whose inhabitants spoke a language closely related to Hebrew, had an almost identical word for the deep and the same conception. The Mesopotamians had the same idea but a different word, the Apsu. They visualised the earth as consisting of three levels: the inhabited surface, a subsurface part where the dead resided, and the Apsu below that, the source of all springs, marshes and rivers (Seely 1997, Horowitz 1998). The land formed a roof over the Apsu. Scholars tend to assume that the Canaanites and the Israelites got their picture of the world from the Mesopotamians, by a process of cultural diffusion. More probably – since the picture is not such as one would deduce from mere observation – the fundamental elements of their cosmology derived from a tradition common to all.
The Earth’s atmosphere is necessary for life. It absorbs harmful gamma- and X-ray radiation from the Sun and other parts of the universe. Its pressure on the surface allows water to exist as a liquid. Along with bacteria, plants and fungi, wind and weather erode the rocky surface and break it down into soil. Oxygen provides animals with the fuel needed to generate energy. Much of the Sun’s heat is re-radiated back into space, but greenhouse gases – dominantly water vapour – trap some of the heat. Without them Earth would be more than 30° colder.
The present atmosphere is divided into four layers: the troposphere at the bottom, where most of the clouds occur and the air convects; the stratosphere, where airflow is mainly horizontal; the temperature-defined mesosphere; and above 80 km the extremely thin thermosphere, the level at which aurorae are generated. The troposphere is 78% nitrogen, 21% oxygen, and 1% other gases.
We know nothing at all about the antediluvian atmosphere. It may have been thicker, and would certainly have had a different composition. Oxygen is the third most abundant element in the universe and the most abundant element in Earth’s mantle and crust, but because of its reactivity it normally occurs only in combination with other elements. One exception is where UV radiation dissociates oxygen from gases such as sulphur/carbon dioxide (Wallner et al. 2022). Another, and much more significant, is where organisms release the element through photosynthesis. Since the first air-breathing animals could not have waited for plants to build up oxygen levels, the oxygen originally in the atmosphere must have been created. Subsequently, plants topped up what was already there, counteracting the processes of weathering and respiration which continually consumed the gas. Without such photosynthesisers we would asphyxiate as well as starve.
After the Deluge, marine bacteria became the main oxygen producers. Although scientists have always assumed that oxygen levels before the Cambrian period were negligible, conditions for the proliferation of marine bacteria were ideal (warm surface waters, abundance of dissolved nutrients), and reports of ‘oxygen oases’ in the literature repeatedly challenge such a picture. In the Carboniferous period, great coal-producing forests boosted oxygen levels up to 30–35%, promoting unusually large body sizes (e.g. dragonflies with 70 cm wingspans) and widespread fires. In the Triassic the proportion dipped below 15%. Owing to much higher rates of volcanism, the atmosphere before the Triassic also had much more carbon dioxide than it does today, keeping the planet warm at a time when heat flow from the Sun was much lower.
Earth’s hot outer core is mostly iron. Convection currents in the core generate electric currents, which generate a giant all-enveloping magnetic field. This magnetosphere protects the atmosphere from erosion by the solar wind and from spasmodic blasts of solar matter known as coronal mass ejections. It also shields terrestrial life from cosmic rays. The field varies over time both in intensity and orientation, and every so often the poles flip, north becoming south and south becoming north. Over the past 200 years the field has weakened by about 9%, and currently magnetic north is moving across the surface at around 44 km per year. Evidence of a magnetic field at least half its present strength goes back a long way, to 3.7 Ga ago in geological time (Nichols et al. 2024). Originally there was no metallic core and no magnetic field; the envelope of water round the solar system provided protection enough.
Creation is not a gratuitous short-cutting of what might have happened anyway were the laws of nature allowed to run their course. Like a battery left in a garage, the universe is running down, as its entropy relentlessly and irreversibly increases. Creation was the bringing into existence of the lowest-entropy state that could have led by natural processes to the present state. Since the highly structured uppermost Earth could not have come about by itself, it was low-entropy. Beneath the deep, the interior was of homogeneous composition, because the processes of melting and mineral segregation that resulted in the planet’s differentiating into crust, mantle and core were such as happen naturally. Thus the interior was also low-entropy, even though it later became layered and in that sense more ordered than the initial state.
At the surface, temperatures were suitable for life. Further down they would have increased as a function of pressure, along what is called the “adiabatic gradient”. If we assume that the gradient below the deep was around 0.3 °C per km (similar to the present mantle), the temperature at the centre would have been around 2,000 °C, as against an estimated present value of 5,000–6,000 °C. The interior would have been solid, melting later as a result of thermonuclear fusion. After reaching peak temperature some time in the Archaean, it slowly cooled towards its present solid state. Thus the mantle was once much more mobile than now and, in the regions where tectonic plates were either subducting or pulling apart, degassing of CO2 was much more vigorous. Even at its present temperature, long-term the mantle behaves like a convecting fluid. Apart from pockets near the surface where the pressure is low enough for it to melt and occasionally erupt, only the outer core is now liquid. The inner core is solid because it is under greater pressure and therefore denser.
Thermonuclear fusion – now occurring only in the centre of stars – was able to occur far down in the Earth’s interior because the speed of light was once much higher. The process generated new isotopes, many of them unstable, and released a vast amount of heat. As the unstable isotopes decayed, they themselves emitted heat. Nuclear fission may also have played a role (Hollenbach & Herndon 2001). The solid interior therefore liquefied, from the centre outwards, and began to differentiate into the chemically distinct regions of inner mantle and core as minerals with lower melting points separated out and denser minerals sank. Temperatures ceased to rise as (1) continuing decline in the speed of light brought thermonuclear fusion to an end, (2) the rate at which the unstable elements decayed into stable, non-radioactive elements declined, and (3) the amount of the radioactive material remaining declined. Although cooler than it was, the present interior is still giving out more heat than it generates. Indeed recent estimates of the heatflow from core to mantle indicate it is cooling faster than was supposed (Murakami et al. 2022). In consequence, plate tectonics must have been decelerating faster than supposed, since it is kept going by the mantle’s convective motions, and these are temperature-dependent.
In addition, the present mantle is 60 °C hotter than supposed (Sarafian et al. 2017), and experiments have shown that the mantle can melt at temperatures 200 to 250 °C lower (Andrault et al. 2018) – a big difference. The presence of CO2, most of which has since outgassed and been captured as carbonate rock, would also have lowered melting temperatures. It can no longer be argued that cycles of ocean crust production and subduction would have required millions of years on the grounds that the subducted slabs had to pass through solid rock. The mantle is not far from liquidus even now.
The oldest minerals known are tiny crystals of zircon and date to just after the Deluge. They show that melting and crystallisation were taking place in the presence of water. The mention in Genesis of gold, iron, copper and quartz (onyx) also implies magmatic processes. Ores form as hot fluids saturated in metal-bearing minerals rise to the surface, cool and precipitate. The heat and pressure involved are not likely to have built up until long into the antediluvian period.
The Earth was the only planet at creation to have had water so far as we know. After the Deluge the water either escaped to the surface or was locked up in the minerals of the upper mantle. The lower mantle appears to have been dry, a dichotomy difficult to account for in the nebula hypothesis. The get-out is to postulate that the first 85% of Earth accreted from already-differentiated planetesimals in the inner disc, after which the source switched to water-rich carbonaceous asteroids from beyond Jupiter (Liu et al. 2023).
Estimates of how much water remains locked in the mantle are steadily improving. Not very long ago it used to be thought there was almost none. Now, as a result of analysing diamonds (which come from deep down in the earth), geologists infer that there is at least as much as at the surface, mostly stored in the transition zone between the upper and lower mantle, 410–660 km beneath the surface (Keppler 2014). Some water enters the interior by being entrained with oceanic plates as they subduct. As they descend, the plates heat up, turning the water into steam which then rises and outgasses along volcanic arcs. Some of the water in the transition zone may be more primeval. The peoples of the Ancient Near East were not entirely wrong in supposing that the great deep still existed.
Because the old landmass was totally destroyed, the names of the places mentioned in Genesis bear no relation to modern geography and hence are themselves evidence that the tradition is authentic. Not even the oldest rocks go back that far, whether exhumed at the surface or buried deep below it. A map of the world would have looked nothing like a modern map.
The first place name in Genesis is Eden, meaning ‘delight’, a byword for a land of fruitfulness and where God planted a garden. Eden was also the name of a locality in the present world (Isa 37:12 and Ezek 27:23), but its location has never been convincingly identified. Genesis tells us that a river flowed up from underground to water the garden and then divided into four. This should be a clue if the world in which Eden was situated still existed, for the rivers are named, and two of them are known: Tigris and Euphrates. Rivers of that name run through present-day Iraq, with headwaters in eastern Turkey, and there have been attempts to show that the other two primeval rivers, Pishon and Gihon, also still exist (e.g. Rohl 1998). However, there is no single river from which the Tigris, Euphrates and two other rivers branch off.
Pishon, moreover, was said to flow ‘round the whole land of Havilah’. Havilah is mentioned in Genesis 10 and 25, where the contexts show that it must have lain in southern Arabia. Since no river connects Turkey with Arabia, it seems safe to conclude that the primeval Havilah was a different country altogether. Gihon in the modern world is the spring on the eastern slope of ancient Jerusalem.
Similar considerations apply to the lands of Cush (Gen 2:13), Assyria (2:14) and Nod (4:16). In Old Testament times Cush was the eponymous name for northern Sudan (Cush being the ‘son’ of Ham whose descendants settled that part of Africa). No river flows all around it. Assyria (Asshur in Akkadian and Hebrew) was a city in central Mesopotamia and, later, a region of which the city was the capital. The Tigris flowed, not east of it as in the antediluvian world, but through it. Nod, to judge from the lack of mention in any other ancient text, had no post-Deluge counterpart. But then it had no Edenic connotations, being associated with an exiled fratricide.
The apparent persistence of topographical names from the antediluvian world is therefore a red herring. While scholars conclude from the impossibility of locating the original Eden that Eden was a myth, the more likely explanation is that the names were re-assigned to the Near East by migrants who knew about such places from the traditions of their forefathers and wished to simulate continuity with that lost world, much as colonists arriving in North America from England sought to recreate what they called the old world with names such as Portsmouth, Cambridge and Boston. Mesopotamia, a land of well-watered plains, of abundant game, cereals, date-palms and pulses, evoked hopes that it might become a new Eden, and its two main rivers were named accordingly. Two of Mesopotamia’s most ancient cities were named after antediluvian patriarchs: Eridu (Eriduk in Sumerian) after Irad (Gen 4:18) and Uruk (Unuk) after Enoch, Irad’s father. Asshur may have been another. When, around the end of the Jemdet Nasr period, the Euphrates breached its banks and devastated a number of the cities, people likened the disaster to the primeval deluge until eventually poets were describing it in the same terms, even to the extent of casting one of their kings in the role of Noah.
As a clue to the landing place of the Ark, ‘the mountains of Ararat’ are also a red herring. Although the mountains (plural, denoting the rings of an impact crater?) were located in the immediately post-Deluge world, that world was very different from the modern, and they too were susceptible to toponym-transfer. The mountains closest to Mesopotamia were the Zagros range. A country or city in the Zagros called Aratta features in several Sumerian texts of the 3rd millennium (the name actually meant ‘mountain’ in Sumerian). According to the later Gilgamesh epic, which conflated the primeval Deluge and the Mesopotamian flood, the Ark ran aground on Mount ‘Nimush’, possibly Pir Omar Gudrun, near Kirkuk. Jeremiah (51:27) mentions that a kingdom called Ararat formed an alliance with the kings of Minni and Ashkenaz. The context suggests an identification with the similarly named kingdom of Urartu, in Armenia. Although this was not in the Zagros range, the truth may simply be that by the 7th century BC some new region had claimed the distinction. Turkey’s Mount Ararat (Agri Dagh) is a volcano that formed near the end of geological history, in the Pleistocene. Its association with the name seems no older than the 11th century AD.
As with primeval Eden, the quest for the remains of Noah’s Ark on the basis that it might exist anywhere in the Near East is the quest for a chimaera.
The light that originally generated cycles of day and night came principally from a source beyond the solar system, daylight having been created three days before the Sun. What could that light have been? Modern astronomy has reached the point where, looking back in time to the farthest reaches of the universe, we can give a definitive answer. In the beginning there were only quasars, not stars in the modern sense. Consisting initially of pure hydrogen, these massive ultra-bright objects were as bright as entire present-day galaxies. They are the oldest and furthest objects detectible with our telescopes and, contrary to the Big Bang hypothesis, their spectra show that they were synthesising elements heavier than hydrogen while still quasars. It was from these bodies that galaxies arose, as they threw out super-energetic jets and the already dense material atomised into stars. The clouds of dust and gas permeating the arms of galaxies are the remains, after star formation, of what was ejected from the centre.
Since our own Milky Way is also a galaxy, it too must have originated in this manner. In the beginning there was only a quasar. Although it has long since shed most of its mass, a mysterious ‘black hole’ containing its collapsed remains still marks the spot. In this respect the Milky Way is unusual. Its black hole is non-luminous, whereas the black holes at the centre of most galaxies are so massive that the friction of the hot, dense matter spiralling around them produces an enormous blaze of light.
The galaxy has three parts: a stellar halo, a thick disc spreading out from the bulge about the centre of the galaxy, and a thin disc beyond the inner disc. The stellar halo has the shape of a rugby ball, with a radius equal to the disc, and a total mass of at least 1 billion suns. It consists of very old stars, mainly distributed as dwarf galaxies and globular clusters. In these concentrations the density of stars per cubic light year can be hundreds of thousands that of the rest of the galaxy, with the oldest stars almost as old as the Universe itself (that is, as old as the Big Bang in the conventional view). Star formation peaked in the halo around 11.2 Gyr and was extremely rapid (Xiang & Rix 2022), all the more so if in absolute terms the first 5 Gyr of time was only half as long as the last 5 Gyr.
The thick disc started to form around 13 Gyr, about the same time as the halo. According to the usual understanding of how galaxies formed, the earliest stars were massive and, because their interiors were hotter than the less massive stars that followed, they were short-lived. Towards the end of their lives they synthesised elements heavier than hydrogen and helium (called ‘metals’ in astronomy) and exploded, leaving clouds from which, in much greater numbers, second-generation stars condensed. As the first-generation stars no longer exist, their existence is an inference; it could have been the central quasar that generated the metals. Either way, since the metals took time to form, the ratio of metals to hydrogen is a guide to stellar age. Star formation in the thick disc stopped around 8 Gyr.
The thin disc younger and contains the remaining stars, including the Sun. They are variably rich in metals and most of them are distributed in spiral arms that project from the ends of the central bar. Unlike its neighbours the Sun is a created star, predating all other stars. Originally there were just two bodies in our cosmic neighbourhood: the nearby Sun and, many present light-years distant, a quasar. Probably the quasar that spawned the stars of our own galaxy was the offspring of an earlier quasar that split into two, one producing the Milky Way and the other Andromeda, the similarly sized galaxy next door to us.
So there were two main phases. In the first, the nucleus expanded in every direction, producing the thick inner disc and ejecting globules of dense gas that condensed into star clusters and dwarf galaxies above and below the disc. Possibly the nucleus collapsed at the end of this phase, around 8 Gyr. Just before the collapse, spurts of metal-rich gas shot out from the nucleus to form the arms, the beginning of the second phase. Here, in the outer disc, the ejected material continued to generate stars. At a much slower rate it still does. The older of these have radial outward motions rather than orbiting about the centre; the younger ones are migrating inward.
Although the galaxy is calculated to have a mass equal to 160–320 billion suns, its visible mass – stars, gas and dust – accounts for only 65 billion suns. Much if not all of the balance is accounted for by a spherical halo of gas that encloses the whole galaxy and extends far beyond it (Nicastro et al. 2016). Presumably it is related to the stellar halo and also originated from the quasar. The temperature of the cloud is not uniform. Part is unusually cool, around 300,000° C, part is around 1.5 million degrees, part around 32 million degrees. How the gas could be so hot after 13 billion, or even 13 million, years is a ‘mystery’ (Das et al. 2021). Other galaxies are also thought to be surrounded by hot gaseous clouds.
The Sun is the only created star and the only one to have started as a concentrated globe of pure hydrogen, the simplest chemical state. Although the quasar initially also consisted of pure hydrogen, it soon began forming other elements, so that, when it later sprayed the space around it with natural stars, these did not consist solely of hydrogen.
The Sun shone from the fourth day onward. As with other stars, the rate at which the Sun fused hydrogen into helium increased over time, accelerated by the heat which gravitational contraction produced (helium being denser than hydrogen) and by the heat from the fusion process itself. With age the Sun got hotter. At the start, its heat output was probably no more than 70% of its present output. Had the rate of CO2 production been no greater than today’s the Earth’s oceans would have been frozen. This is known as the ‘faint young Sun problem’, since geological evidence shows that the oceans were considerably warmer than today. To solve it one has to postulate levels of CO2 possibly as high as 50,000 ppm at the beginning of the geological record (today: 430 ppm). After water vapour, CO2 and SO2 were (are) the main volcanic gases, so atmospheric concentrations of this order are certainly conceivable – but only if undersea volcanism was much more intense. intense. In that case the ocean crust and the water above it would have been much warmer anyway and CO2 concentrations might not have needed to be as high as 50,000 ppm. We should also consider the possibility that the entire solar system was warmer than today, with much of the missing heat and light coming from the primordial quasar. .
Higher rates of radioactive decay were linked to the posited higher velocity of light (‘c’). The decline in c affected the entire universe and must itself have had a physical cause, possibly to do with the energy of the so-called vacuum.
The Moon was created on the fourth day of Creation, its stated purpose being to divide the year into months and alleviate night’s darkness. Unstated purposes would have included the stabilisation of the Earth’s axial tilt and the production of tides.
Amongst those looking for a natural explanation the leading idea for the past three decades has been the hypothesis that Earth collided with a smaller planet that disintegrated and partly vaporised in the collision. Most of the debris from which the Moon then formed came from the impactor. Since all planets differ in isotopic composition, the hypothesis predicts that the Earth and the Moon will also differ in composition. In fact, the isotopic signatures of the Earth and the Moon are remarkably similar. According to Linda Elkins-Tanton (2013), the Giant Impact Hypothesis, as it is called, is in crisis and ‘creative thinkers’ are required to make it plausible. One more recent suggestion, generally regarded as unsatisfactory, is that the Moon formed from a succession of smaller debris-forming impacts (Rufu et al. 2017); another is that it formed from a collision with another planet directly (Kegerreis et al. 2022). One can never say never in such circumstances, but, as things stand, the Moon’s ongoing resistance to natural explanations constitutes evidence that the satellite did not have a natural origin, even if scientists themselves will not give up looking and hoping for one.
In itself, the idea of a planet exploding is not necessarily far fetched. The Genesis tradition tells how, early on in history, a hail of celestial objects crashed through ‘the apertures’ and broke up the uppermost layer of the Earth. In the light of modern knowledge, such an event would be consistent with the explosion of a planet somewhere between the orbits of Mars and Jupiter, where asteroidal remnants of the explosion still exist. On the Moon, craters up to thousands of kilometres across represent abiding testimony of the cataclysm.
The surface of the Moon was originally not cratered. Its surface topography of volcanic ‘seas’ and crater-rimming mountains is entirely the effect of bombardment. The vast plains of basalt (maria) that blotch the surface erupted in the wake of the impacts.
The Moon’s upper crust consists mostly of a white igneous rock rich in feldspar, known as anorthosite. It is igneous in the sense that its mineralogy and texture is that of a rock crystallising out of a chemically more primitive magma. The solid state implies an earlier molten state because magma will cool and crystallise by itself to produce the rock, and creator input is not required. Cosmologists are not being unreasonable when they deduce that the crust must have crystallised from a ‘magma ocean’. This low-density iron-depleted rock type is exactly what one would expect at the surface if the interior melted.
So, some time between the Creation and the bombardment, the Moon must have been resurfaced, as a result of the heat released by thermonuclear fusion and the decay of the radioactive elements produced by the fusion. None of the rocks sampled by the Apollo missions go back to the Creation. Magmatism remade the lunar crust, the cooling of which was accelerated by water from outer space – falling at the same rate, presumably, as that which produced shallow oceans on Mars at this time. The indistinct rims of the oldest craters show that the crust was extremely hot and still plastic when the first asteroids fell (Kamata et al. 2015).
The first two laws of thermodynamics are fundamental to everything we know about the universe. The first states that energy, once having been brought into existence, can neither be created nor destroyed. The second encapsulates the truth that the universe is continually becoming less ordered. An initial state that was not one of maximum order would imply an earlier state of greater order, which is excluded by definition. It follows that the original solar system began in a state of maximum order.
The length of the Earth’s year may illustrate the point. The present value of 365¼ days does not reflect a state of maximum order, because it is not a whole number, and even without the fraction, 365 days is intrinsically arbitrary – why not 364 or 366? By contrast, an original value of 360 days would be consistent with a state of maximum order. Being a whole number it would have been unique, and being exactly divisible by 12 it would have been a perfect number. There would have been no implication of its having evolved by degrees from a state that was more ordered than that of the perfect number. The present value of 365¼ days implies that the year has lengthened over time.
Similarly with the length of the month. At present it takes just over 29½ days for the Moon to complete its orbit round the Earth. This non-unique number also does not reflect a state of maximum order. The original number must have been 30 days, with the year consisting of twelve months totalling 360 days. At present the lunar month is lengthening rather than shortening as the distance between Earth and Moon slowly increases.
All these numbers, 360, 12 and 30, imply that the original system of counting was sexagesimal, based on the number 60, rather than decimal. In fact we know that the oldest counting system was sexagesimal, because this was the system of the Sumerians, who founded the world’s first civilisation in the 4th millennium BC. Similarly they divided the circle into 360 degrees and divided the day into 24 hours. Inheriting their mathematics, we still use these divisions.
Now | At first | |
Length of year (days) | 365.24 | 360 |
Length of lunar month (days) | 29.53 | 30 |
Number of months in a year | 12.37 | 12 |
Tilt of the Earth’s axis (degrees) | 22.1-24.5° | 24° |
Tilt of Sun’s axis relative to Earth’s orbit | 7.25° | 7° |
Eccentricity of planetary orbits | 0.007-0.206 | 0 |
Number of planets | 8 | 12 |
Reasoning in this way, we can infer that the original number of planets was 12, not 8, and that they all orbited round the Sun precisely on the plane of the ecliptic (as defined by the Earth’s orbit round the Sun). Today, most planets orbit within 3° of the ecliptic, with Venus orbiting at an angle of 3.4° and Mercury at 7.0°. Likewise, the original tilt of the Earth’s axis relative to the ecliptic would have been 24° rather than the present 23.5°, and the original tilt of the Sun’s axis would have been 7° rather than the present 7.25°. All planetary orbits would have been perfectly circular. Subsequently, as a result of asteroid impacts and the gravitational influence of the planets on each other, they settled into elliptical orbits. Most planetary orbits are almost circular, varying from less than 0.007 (Venus) to 0.05 (Saturn). Mercury’s eccentricity is 0.206 and Mars’s 0.093 (where 0 is perfectly circular and 1 is a parabolic trajectory); the orbits of these planets relative to their mass are highly anomalous.
Genesis’s day-by-day account of the Flood implies that months then were all 30 days (Gen 7:11, 8:3f). During the period between the Creation and the Flood four of the twelve planets exploded, with the explosion nearest the Earth giving rise to the asteroid belt between Mars and Jupiter. Bombardment by fragments from the explosion was probably what disturbed Mars’s orbit. At some later date the Earth and the Moon were also bombarded. The effect was to push the Earth away from the Sun and/or decrease the length of the day, and to push the Moon slightly closer to Earth. So far as solar eclipses were concerned, the two effects were self-cancelling. The Sun was smaller at the Creation than now (since it expands as it burns) but closer to the Earth. The Moon’s distance from the Earth relative to the Earth’s distance from the Sun remains as good as perfect.
Venus and Uranus rotate around their axes in the opposite direction from the other planets, from east to west; Mercury’s spin is also thought to have originally been retrograde. Arguably they were created thus to make it clear that the solar system did not have a natural origin. Equally odd is Uranus’s 98° tilt. A recent study attributes it to a giant impact (Kegerreis et al. 2018), probably about the same time as Uranus captured its moon Triton, the only large moon to orbit its planet backwards.