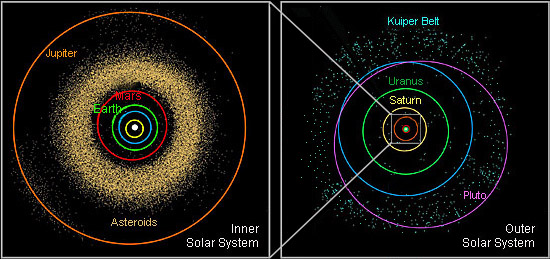
If asteroids began within a degree or two of the ecliptic, low-angle inclinations can be explained by low-energy collisions, which certainly occurred. Impact scars are common even on quite small asteroids. Higher-angle inclinations are more difficult to explain and raise the possibility of an explosive origin. Some of the fragments would sooner or later have crashed into the Sun or other planets, or been captured by other planets to become satellites; some would have settled into synchrony with Jupiter’s orbit. Known as Trojans, these mostly have a D-type spectrum, like the asteroids in the main belt closest to them.
Much of the material presumed to be missing from the belt was swallowed up by Jupiter, which thereby acquired a diffuse rocky core, equal to about 10–30 Earths. (This sounds enormous, but a planet with 25 times Earth’s mass would have had a diameter just 3 times greater, and Jupiter would still have been many times more massive.) In the other direction, much of the material would have ended up in the Sun.
The only way of probing the Sun’s composition is through analysing its atmosphere, on the assumption that the atmosphere is representative of the Sun as a whole. If the star condensed from gas that was already impregnated with metals, its composition, apart from helium and volatiles, should be similar to that of chondrites, which it is. Alternatively, the presence of heavier elements is due to planetary debris plunging into the Sun. In that case too the composition should be chondritic.
The explosion of a large planet between Saturn and Uranus would similarly account for Saturn’s core. Distances in the solar system are measured in astronomical units or AU, one unit being the mean distance between the Sun and the Earth. The orbits of Saturn and Uranus are 9.6 AU apart, the same as between Saturn and the Sun, so there is plenty of space for another planet. Beyond Jupiter are the centaurs, mostly between Saturn and Uranus and possibly numbering several million.
As a population, the existence of centaurs (half man, half horse in Greek mythology) was not known about until the 1990s. They were thought to be half comet, half asteroid. Hidalgo, the first to be discovered, has a spectrum characteristic of D-type asteroids, an eccentric orbit varying from 1.9 to 9.5 AU and an inclination of 42°. Damocles orbits at a distance of 1.6–22 AU and has an inclination of 62°. The largest confirmed centaur, the 260 km Chariklo, orbits between 13.1 and 15.8 AU and has an inclination of 23°. Because of their water content, some main-belt asteroids are thought to have been centaurs drawn sunward by the gravity of Jupiter. Some centaurs may have originated from the Kuiper Belt.
As with every other class of solar system body, ideas about comets have changed. Once assumed to be unaltered leftovers of the cold outer nebula, they have proved to be much the same as asteroids, only richer in volatiles (frozen frozen H2O, CO2, CO, CH4, NH4). We know most about the short-period comets, those with orbits of less than 200 years. Some consist primarily of volatiles; some have solid nuclei, dented by craters; others are low-density rubble piles. They manifest as comets when their elliptical orbits bring them close to the Sun. Each year several asteroids are found to exhibit a coma and/or tail and are reclassified accordingly.
The data gathered by the instruments that probed the short-period comets Tempel 1 and Wild 2 were revelatory. Contrary to the expectation that the silicate particles in them would be amorphous like the dust in interstellar space, the particles turned out to be crystalline, indicating crystallisation from melts at temperatures up to 1700° C (Grossman 2010). Analysis of one such silicate in Wild 2 indicated a cooling rate of 10–100° per hour, in agreement with the rates of chondrules and asteroidal lava (Leroux et al. 2008). Carbonates, iron, CAIs and chondrules were also detected.
The existence of high- and low-temperature materials in the same body was mystifying. Since the core had to have preceded the volatiles around the core, its rocky composition suggested an origin in the inner solar system. On the other hand, the orbits of comets and the abundance of volatiles point to an origin in the outer solar system. Did rocky planets also exist there?
‘Long-period’ comets take more than 200 years to go round the Sun. Their pathways are highly elliptical and eccentric – attributed ad hoc to the gravitational pull of passing stars – and extend far beyond the observable solar system. Like short-period comets, fundamentally they do not differ from asteroids in composition. They can orbit the Sun at any inclination and are as likely to have a retrograde orbit (going in the opposite direction to the planets) as a prograde one. Not unreasonably, the solar system is thought to be enclosed by a vast replenishing reservoir of such comets, known as the Oort Cloud, too far away to be directly observable.
The difference in orbital period between the two types is not absolute. Long-period comets can become short-period if the gravity of the giant planets draws them into the inner solar system. Most short-period comets orbit between Mars and Jupiter and are thought to have drifted in from the Kuiper Belt.
With the exception of Earth’s moon, probably none of the planetary satellites formed in situ. Mercury and Venus have no satellites. Mars has just two, being either asteroids or impact-lofted fragments of the planet itself. Jupiter has 92 confirmed and Saturn 146, the majority less than 10 km in diameter. Uranus has 28, Neptune 16. Once captured, the different bodies frequently crashed into each other, sometimes resulting in complete fragmentation. Occasionally the fragments turned into rings, or re-assembled to form new moons. Other collisions left the moons cratered and scarred. Spherical shape is not evidence of creation, since above a certain mass self-gravity will naturally pull a body into a sphere.
Astronomers classify the satellites into regular irregular. Regular satellites trace close, circular orbits about the host’s equator, orbit in the same direction as the planet’s rotation, and are supposed to have formed from the same material and at the same time as their hosts. However, while the moons are predominantly rocky, the hosts are predominantly gaseous. Irregular satellites trace more distant, elliptical paths at an angle to the equator. Most satellites are irregulars, with high orbital inclinations and eccentricities.
At 2700 km diameter, Triton is by far the biggest of Neptune’s moons, has a steeply inclined but circular retrograde orbit, and lies between a group of inner prograde satellites and several outer satellites with both types of orbit. Density calculations suggest that its composition is similar to Pluto’s. It is larger than Pluto and almost certainly originated from the Kuiper Belt.
The present solar system offers no mechanism by which moons could be captured, so the thinking is that captures must have taken place early in its history, when collisions and near misses were more likely (Jewitt et al. 2006).